
Expired activity
Please go to the PowerPak
homepage and select a course.
Emerging Rapid-acting Insulin Therapies
INTRODUCTION
Diabetes is a common disease and its incidence continues to grow in the United States (U.S.) and other developed countries. Coincident with this growth, the pharmacologic management of diabetes has evolved dramatically in recent decades.1 Much of the expansion in the pharmacologic armamentarium in the past 20 years has been due to the introduction of non-insulin therapeutic agents, including thiazolidinediones, meglitinides, glucagon-like peptide-1 (GLP-1) receptor agonists, dipeptidyl peptidase-4 (DPP-4) inhibitors, and sodium-glucose transporter-2 (SGLT-2) inhibitors. During the same timeframe, insulin therapy has also evolved. The first rapid-acting insulin analog (RAIA) was approved for use in 1996. This was followed by 2 other RAIAs and several long-acting insulin analogs. This manuscript will provide a brief overview of the epidemiology and pathophysiology of diabetes, as well as the costs associated with the management and monitoring of diabetes. The primary focus of the manuscript will be the pharmacology and clinical use of RAIAs. Novel insulin delivery platforms and formulations will also be presented.
EPIDEMIOLOGY, PATHOPHYSIOLOGY, AND COSTS OF DIABETES
The fraction of the U.S. population and the absolute number of individuals with diabetes have burgeoned in recent decades. In addition to the increased incidence of the disease, the health care costs and complications associated with diabetes are significant and are of grave concern. In 2014, an estimated 22 million adults and children in the U.S. had a diagnosis of diabetes.2 This is 4 times higher than the number of people diagnosed with diabetes in 1980. However, despite the sophistication of our health care system and the high profile of diabetes in the news and the press, many people with the disease remain undiagnosed. In 2012, the American Diabetes Association (ADA) estimated that approximately 29 million people in the U.S. had diabetes; of these, more than 8 million were believed to be undiagnosed.3 Type 1 diabetes accounted for approximately 1.25 million cases of the disease in 2012; the overwhelming majority of individuals with diabetes have type 2 disease. Additionally, approximately 86 million people were estimated to have prediabetes in 2012, which represents an increase of 7 million people since 2010. Approximately 1.4 million new cases of diabetes are diagnosed every year in the U.S.3
The damaging effects of chronic, prolonged hyperglycemia are frequent and debilitating, and diabetes was estimated to be the 7th leading cause of death in the U.S. in 2010. The overall risk of death for a patient with diabetes is approximately twice that of a person without diabetes of a similar age. In addition to an increase in mortality rates, patients with diabetes also suffer a high incidence of associated morbidity as a result of complications of both the disease and its treatment. After adjusting for age differences, the rate of death due to cardiovascular disease was approximately 1.7 times higher in patients with diabetes than in patients without diabetes aged 18 years or older. Additionally, rates of myocardial infarction were 1.8 times higher and the rates of hospitalization for stroke were 1.5 times higher in people with diabetes aged 20 years or older. Diabetes is the leading cause of blindness in working-aged Americans and, between 2005 and 2008, approximately 29% of people older than 40 years had evidence of diabetic retinopathy. In 2011, diabetes was the leading cause of chronic renal failure (44%) in all new cases of renal failure. Diabetes is also the leading cause (60%) of non-traumatic amputations in the U.S. In 2010, approximately 73,000 patients with diabetes required a lower-limb amputation. Patients with diabetes also frequently present with hyperlipidemia and/or hypertension. From 2009 to 2012, 71% of patients 18 years or older with diabetes also had hypertension (blood pressure ≥ 140/90 mmHg or taking anti-hypertensives) and 65% had hyperlipidemia.3
In some cases, the management of diabetes may have deleterious effects on patients. In 2011, approximately 282,000 patients aged 18 years or older who had a diagnosis of diabetes received care in an emergency department for the management of hypoglycemia.3 The sequelae secondary to hypoglycemia may range from mild symptoms to death.4
The total cost of diabetes incurred by the health care system is inordinately high and, on an individual basis, health care costs are estimated to be 2.3 times higher for people with diabetes than for age- and gender-matched individuals without diabetes.3 In 2013, the total cost of diabetes in the U.S. was estimated to be $245 billion dollars, including $176 billion dollars in direct medical costs and $69 billion of lost productivity.
Type 1 diabetes tends to present in younger individuals (< 35 years of age), but it may occur at any time.5 The current theory of the etiology of type 1 diabetes is that a genetically predisposed individual is affected by a precipitating event or factor. Proposed precipitating factors have been the subject of debate and include viruses, bacteria, milk, wheat, and other substances.5 It appears that after exposure to some precipitating factor, under the right conditions, the body mounts an immunologic attack on the beta-cells of the pancreas. Over time, beta-cell function declines and, eventually, it declines to a point where overt diabetes is present.
Type 2 diabetes has historically been a disease that primarily affected adults and, because of this, it was previously known as adult-onset diabetes mellitus. However, this pattern has changed dramatically during the past few decades, and young adults and children are now frequently diagnosed with type 2 diabetes.3 Recent reports indicate that approximately 21% of newly diagnosed cases of diabetes among U.S. children and adolescents are type 2 disease.
The pathogenesis of type 2 diabetes is complex. The development of hyperglycemia secondary to type 2 disease occurs under conditions of insulin resistance overlaid with a relative reduction in insulin response.6 In many, but not all, cases, insulin resistance is driven by obesity. Initially, obese patients are hyperinsulinemic, but, over time, beta-cell function and mass are reduced. In the presence of continued insulin resistance, obese individuals eventually become hyperglycemic. The loss of beta-cell function is progressive and continues with the disease. Patients with type 2 diabetes are either insulinopenic or are relatively insulinopenic (i.e., they do not secrete enough insulin to mitigate chronic hyperglycemia).6
GOALS, MONITORING, AND POSTPRANDIAL HYPERGLYCEMIA
There are several published guidelines that provide glycemic goals and recommend monitoring parameters for patients with diabetes.7,8 This monograph will focus on the recommendations of the ADA.
In most cases, an assessment of glycemic control includes an evaluation of a patient's hemoglobin A1c (A1C) level and the self-monitoring of blood glucose levels.8 Some patients may be appropriate candidates for the supplemental use of continuous glucose monitoring, which measures interstitial glucose levels.
Glycemic goals for most non-pregnant adults with diabetes include an A1C level of less than 7%, a preprandial plasma glucose level of between 80 and 130 mg/dL, and a peak postprandial plasma glucose concentration of less than 180 mg/dL. Generally, postprandial glucose levels should be included in a patient's testing regimen when A1C goals are not met after preprandial goals have been reached.
The recommended glycemic goals are reasonable for the "average" patient, but each patient's goals should be individualized. Some patients may be better suited for more stringent goals while others require less stringent goals. Considerations for establishing goals include the risks associated with hypoglycemia and other adverse drug events; disease duration; life expectancy; other comorbidities; existing vascular complications; the patient's perspective, attitudes, and expectations; and the resources and support systems available to the patient.8 For example, an 87-year-old patient with a 1-year history of type 2 diabetes with a history of hip fracture due to dizziness and instability would likely experience better outcomes with less stringent glycemic goals.
Postprandial glycemia contributes significantly to overall glycemic exposure.9,10 A study in 2003 suggested that the overall contribution of fasting/pre-meal hyperglycemia was greater than that of postprandial hyperglycemia in patients with higher A1C values.9 Specifically, at A1C values greater than 10.2%, the relative contributions of fasting/pre-meal basal and postprandial glucose levels to overall glycemic exposure were approximately 70% and 30%, respectively. This pattern was reversed in patients with A1C levels lower than 7.3%: the relative contributions of fasting/pre-meal basal and postprandial glucose levels were approximately 30% and 70%, respectively. This suggested that the contribution of postprandial glycemia to overall glycemic exposure was more significant at lower A1C levels.
A more recent study reported that the contributions of postprandial and fasting/pre-meal basal glucose levels to overall glycemic exposure were 22% and 78%, respectively, at baseline and were not contingent on A1C.10 After approximately 4 weeks of therapy, the relative contributions of postprandial and fasting/pre-meal basal glucose to the overall glycemic load had changed to 66% and 34%, respectively, in the basal insulin treatment group and to 32% and 68%, respectively, in the insulin lispro, premixed insulin, and oral anti-diabetic intensification groups. The authors did not observe a relationship between A1C and the relative contributions of postprandial and basal glucose to overall glycemic load in these groups after 4 weeks of treatment. They concluded that the form of treatment used by patients can be a more significant factor than A1C in determining the relative contributions of postprandial and basal glucose to overall glycemic exposure.
Taken together, these studies, along with other trials not cited, suggest that both postprandial and basal glucose levels are significant contributors to overall glycemic exposure and, therefore, glucose toxicity. Considering this, it is incumbent on health care providers who manage hyperglycemia in patients with diabetes to remain mindful of the impact of not only fasting or pre-meal glucose levels but also postprandial glucose levels on A1C. These interrelated factors – pre-meal and post-meal glucose – are significant markers of glycemia and of the patient's overall exposure to the harmful effects of hyperglycemia.
MANAGEMENT OF HYPERGLYCEMIA WITH INSULIN IN PATIENTS WITH TYPE 1 DIABETES
The ADA recommends 4 principles that should be included in the management of hyperglycemia in patients with type 1 diabetes.11 First, insulin should be given via a multi-dose regimen that includes 3 to 4 injections per day of basal and prandial insulin or via a continuous subcutaneous insulin infusion pump. Second, prandial insulin doses should be calculated on the basis of the insulin-to-carbohydrate intake, the pre-meal glucose level, and any anticipated physical activity. Third, most patients should be treated with insulin analogs, particularly those who are at greater risk of hypoglycemia. Fourth, a sensor-augmented low glucose threshold suspend pump should be considered for patients with frequent nocturnal hypoglycemia or recurrent severe hypoglycemia and for those with hypoglycemic unawareness.
The total daily insulin dose requirement for a patient must be individualized, but, generally, approximately 0.5 to 0.6 units of insulin per kg of body weight are required for a patient with type 1 diabetes. For example, an individual who weighs 70 kg would require 35 to 42 (70 kg × 0.5 to 70 kg × 0.6) units of insulin daily.12 Approximately one-half of this dose would be given as a basal insulin analog, and the remaining half would be administered as an RAIA and would be divided among the 3 meals of the day. The 3 meal-time doses might not be equal because they would be contingent on factors such as the patient's dining habits. The titration and dose adjustment of prandial insulin will be discussed in the RAIA dosing concepts section.
MANAGEMENT OF HYPERGLYCEMIA WITH INSULIN IN PATIENTS WITH TYPE 2 DIABETES
The vast majority of patients with type 2 diabetes will be managed initially with metformin unless it is contraindicated or not tolerated.11 If a patient does not achieve his or her glycemic goals with metformin monotherapy, another oral agent may be added to metformin such as a sulfonylurea, thiazolidinedione, DPP-4 inhibitor, or SGLT-2 inhibitor. Alternatively, a GLP-1 receptor agonist or basal insulin may be added to metformin. Newly diagnosed patients may be treated with insulin initially if they are markedly symptomatic or have extremely high glycemic indices at the time of presentation.
When insulin is initiated in a patient with type 2 diabetes, the first step is usually a basal insulin such as glargine, degludec, or detemir. The basal insulin dose is titrated on the basis of the patient's fasting glucose level and the glycemic target. Generally, a starting dose of 10 units or 0.1 to 0.2 units/kg/day is used. If the patient's A1C is not at goal after the fasting glucose goal has been achieved or after a basal dose of greater than 0.5 units/kg/day has been reached, then the addition of a GLP-1 receptor agonist or prandial insulin should be considered. If insulin is appropriate, a single dose of rapid-acting insulin with the largest meal of the day or twice-daily premixed insulin could be considered. If twice-daily premixed insulin is initiated, the basal insulin should be discontinued.
COMPARISON OF INSULINS
Historically, animal-source insulins were widely used, but, today, the insulins primarily in use are recombinant human insulins or recombinantly produced analogs of human insulin. Prior to 1983, all insulin preparations were derived from animal sources (primarily beef and pork). In 1983, the first recombinant medication, human insulin, was approved. The primary clinical problems with conventional human and animal-source insulins were related to the fact that the pharmacokinetic (PK) and pharmacodynamic (PD) profiles of the available insulins did not approximate normal physiologic insulin secretory and glucose-lowering profiles. This prompted searches for analog insulins, including "flat," slow-release basal insulins and rapid-acting insulins that more closely resemble normal physiologic insulin secretory patterns. Insulins with slow, constant release over a long time interval that mimic basal secretory patterns and insulins with very rapid absorption and rapid elimination that mimic prandial insulin secretory patterns have been developed. The first rapid-acting human insulin analog (lispro) was approved in 1996. This was followed over the next 20 years with a succession of additional novel insulin analogs, including rapid-acting insulin aspart and insulin glulisine and long-acting basal analogs insulin glargine, insulin detemir, and insulin degludec.1
These highly pure insulin analogs, both long-acting and rapid-acting, are produced in virtually unlimited quantities via recombinant technology. The amino acid sequences of all currently available insulin analogs are intentionally altered to enhance a specific desired PK characteristic but retain affinity and activity at the level of the insulin receptor.12,13 Table 1 provides a comparison of the PK properties of the insulin analogs that are currently on the market.
Table 1: Pharmacokinetic properties of insulin preparations12-14 |
Insulin(s) |
Onset of action |
Peak activity |
Duration of action |
Lispro, aspart, glulisine |
5 to 15 min |
1 to 2 hr |
4 to 6 hr |
Human regular |
30 to 60 min |
2 to 4 hr |
6 to 10 hr |
Human NPH |
1 to 2 hr |
4 to 6 hr |
10 to 16 hr |
Detemir |
- |
None |
Up to 24 hr |
Glargine U-100 |
- |
None |
Up to 24 hr |
Glargine U-300 |
- |
None |
> 24 hr |
Degludec U-100, U-200 |
- |
None |
> 24 hr |
Structure and formulation of rapid-acting analogs
Pharmaceutical human insulin has an affinity for itself and is found in the monomeric form only when insulin is in low concentrations. In concentrated solutions, insulin molecules tend to aggregate together to form pairs or dimers that, in turn, aggregate into hexamers composed of 6 molecules of insulin.14,15 The presence of zinc, which is used to stabilize insulin, favors the formation of hexamers even more. When injected, hexameric insulin slowly disassociates into dimers and then into monomers, which can cross the subcutaneous surface and enter circulation. This process is a slow, rate-limiting step that requires 30 to 60 minutes before significant insulin activity is observed. Because of this slow onset of activity, regular human insulin is typically administered 30 to 45 minutes prior to a meal. Peak concentrations of insulin after a subcutaneous injection of regular human insulin occur 2 to 4 hours after the injection and the effects may persist for up to 10 hours. This PK/PD profile does not approximate normal prandial physiologic secretory patterns and lends itself to less-than-adequate postprandial coverage in the first hour after a meal, as well as hypoglycemia prior to the next meal. Rapid-acting human insulin analogs were developed to mitigate these problems.
Currently, there are 3 RAIAs on the market in the U.S.: insulin lispro, insulin aspart, and insulin glulisine.12 Each of these insulin analog molecules has been slightly modified from native human insulin (Figure 1). Insulin lispro is identical to human insulin with the exception of the juxtaposition of proline and lysine at positions B28 and B29. Insulin aspart utilizes a single substitution of aspartic acid for proline at position B28. The modifications in insulin glulisine include the substitutions of lysine for asparagine at position B3 and glutamic acid for lysine at position B29.
Figure 1: Molecular structures of insulin lispro, insulin aspart, and insulin glulisine15
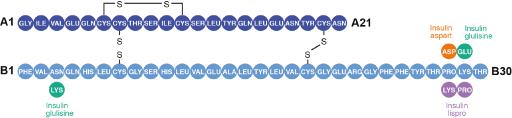
Reproduced with permission from Reference 15.
These changes are located toward the end of insulin's B chain and serve to destabilize or reduce the formation of hexamers but retain insulin receptor affinity and activity. Because of these changes, RAIAs tend to exist in the monomeric form and are absorbed more rapidly than regular human insulin.15 In addition to the altered molecular structures, the formulations of these insulins also impact their PK/PD profiles.15 Insulin lispro and insulin aspart formulations both contain glycerin, metacresol, zinc, phenol, and disodium hydrogen phosphate. The formulation of insulin glulisine is slightly different: it does not contain zinc, but it does contain metacresol, tromethamine, sodium chloride, and polysorbate 20.
PK and PD profiles of rapid-acting analogs
The PK and PD profiles of the 3 RAIAs are comparable. Three similar, but not identical, trials in patients with type 1 diabetes compared the PK and PD profiles of insulin lispro, insulin glulisine, and insulin aspart to those of regular human insulin (Figure 2). The first study compared the impacts of insulin lispro and regular human insulin on the glycemic excursion secondary to a carbohydrate-rich meal in 10 patients with type 1 diabetes.16 Either insulin lispro or regular human insulin was injected just prior to the meal. After the insulin lispro injection, the post-meal area under the blood glucose curve was 12% less than was observed after the regular human insulin injection. Peak plasma glucose levels occurred at 41 minutes after regular human insulin and 37 minutes after insulin lispro administration; levels were 17% lower after insulin lispro administration. The study concluded that insulin lispro provided superior postprandial glucose coverage compared to regular human insulin.
The second study compared insulin aspart to regular human insulin after a meal in 22 patients with type 1 diabetes.17 In this trial, insulin aspart was injected just before the meal, regular human insulin was injected 30 minutes before the meal, or regular human insulin was injected just before the meal. The mitigation of the glycemic excursion as measured by the glucose area under the curve with insulin aspart (891 mmol x min/L) was superior to regular human insulin injected just before the meal (1311 mmol x min/L) and to regular human insulin injected 30 minutes before the meal (1106 mmol x min/L). The mean maximum glucose concentration was approximately 18% lower after insulin aspart than after regular human insulin injected immediately before the meal. Insulin apart was absorbed approximately twice as fast as the regular human insulin. The study concluded that insulin aspart provided superior postprandial glycemic control compared to regular human insulin.
The third study was a 4-way cross-over trial that compared insulin glulisine to regular human insulin after a standardized meal in 20 patients with type 1 diabetes.18 The study conditions included insulin glulisine injected immediately before the meal or 15 minutes after the meal and regular human insulin injected 30 minutes before the meal or immediately after the meal. Insulin glulisine administered immediately before the meal resulted in lower maximum glucose excursions than were observed with immediate pre-meal regular human insulin (65 vs 89 mg/dL). Mean maximum glucose concentrations with these 2 conditions were 180 and 209 mg/dL, respectively. The time to maximal glucose concentration was also reduced with insulin glulisine compared to regular human insulin (55 vs 97 minutes). Peak concentrations of insulin glulisine were double those observed with regular human insulin and occurred in half the time. The PD effects (with respect to glycemic indices in the study) of insulin glulisine injected 15 minutes after the meal were similar to those observed with regular human insulin injected 30 minutes before the meal. This study concluded that insulin glulisine provides postprandial glucose disposal that is closer to normal physiological patterns than regular human insulin. The study also concluded that insulin glulisine enables appropriate timing of postprandial insulin.
Figure 2. The pharmacokinetics of rapid‐acting insulin analogs and their clinical consequences15
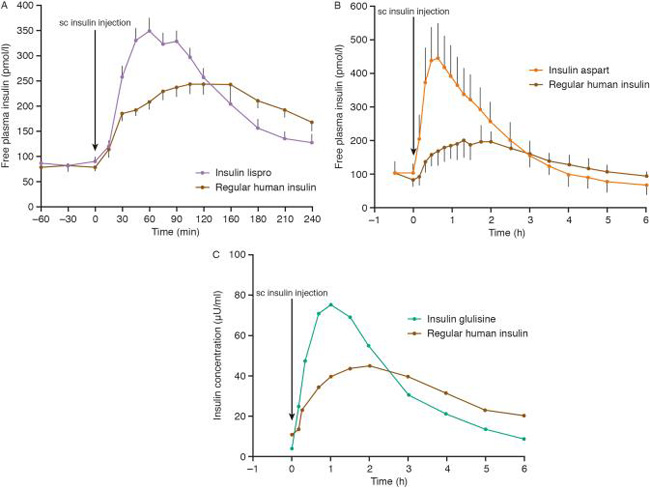
Reproduced with permission from Reference 15.
In addition to differences in molecular structure and formulation, the location of the subcutaneous injection site also affects the PK properties of insulin and insulin analogs. Subcutaneous abdominal injection provides the most rapid and predictable absorption patterns, likely because of the overall local circulation and the higher body heat in that region.12 The arm, thigh, and buttocks are other anatomical regions that may be appropriate for injection, but they provide slower absorption patterns than the abdomen.15
While the PK and PD profiles of the 3 RAIAs are similar, their package inserts suggest different ranges of administration times.19,20,21 Insulin lispro should be injected within 15 minutes before a meal or immediately after a meal; insulin aspart should be given within 5 to 10 minutes before the start of a meal; and insulin glulisine should be administered between 15 minutes before and 20 minutes after the initiation of a meal. European guidelines are simpler and state that RAIAs should be administered shortly before or soon after a meal.15 One of the benefits of the RAIAs is that they can be administered just before the meal rather than in a staggered fashion, as is the case with regular human insulin. This provides a level of convenience that does not accompany regular human insulin, which should, in most cases, be injected 30 minutes prior to a meal in order to attain reasonable postprandial insulin levels and glucose control.
A recent review of the 3 RAIAs reinforced findings that the PK and PD profiles were similar despite slight differences among the insulins. Specifically, the review stated that insulin glulisine had a faster onset of action than the other 2 analogs but that this difference had not been shown to be of clinical significance in terms of treatment satisfaction, reduced rates of hypoglycemia, or glycemic control.15
RAIA DOSING CONCEPTS
There are a number of methods that can be employed to calculate and/or adjust the dose of an RAIA. One simple method of initiating a dose of rapid-acting insulin with the largest meal of the day in an adult patient with type 2 diabetes is to simply begin with either 4 units, 0.1 units/kg, or 10% of the basal dose.11 The basal dose should be reduced by the same amount as the added dose of rapid-acting insulin if the A1C level is less than 8%. The RAIA dose can be slowly titrated by 1 to 2 units (or by 10% to 15%) 2 times a week until the self-monitoring blood glucose goals are attained. If hypoglycemia occurs and the cause is determined to be the insulin, then the dose should be reduced accordingly. If a second or third dose of an RAIA is to be added to the patient's regimen, the same process may be used. Overall, this method of initiating and titrating insulin doses is static, but patients can use this simple method in combination with rules for adjustment based on the size and content of a meal and expected physical activity.
More dynamic and proactive methods to determine an RAIA dose prior to each meal are commonly used by patients with type 1 diabetes, but they may also be used by patients with type 2 diabetes. Generally, 1 unit of insulin will cover the glycemic excursion that results from the consumption of 10 to 15 grams of carbohydrates in a patient with type 1 diabetes.12 If a patient knows the number of grams of carbohydrates that are contained in a meal, he or she can determine the dose of rapid-acting insulin needed to cover that meal. This method must be individualized, and this individualization is often accomplished by use of the insulin-to-carbohydrate ratio.12 This ratio indicates the number of grams of carbohydrate that are covered by 1 unit of insulin. The typical insulin-to-carbohydrate ratio ranges from 1:10 to 1:15 and it is ascertained for a particular patient through observation and experience. The ratio also varies with different food types and with different meals. For example, if a patient has an insulin-to-carbohydrate ratio of 1:10 and he is preparing to consume a meal that contains 60 grams of carbohydrates, he would need 6 units (1 unit for every 10 grams of carbohydrates) of rapid-acting insulin to cover the glucose excursion secondary to that meal. (This method assumes that the pre-meal glucose level is within the target range. If the pre-meal glucose is high, then an adjustment may be required.)
In another example, a patient has an insulin-to-carbohydrate ratio of 1:15 and she also knows on the basis of her experience that 1 unit will lower her glucose approximately 50 mg/dL. Prior to a meal, the patient's glucose is 100 mg/dL over the pre-meal target and she is going to consume a meal that contains 60 grams of carbohydrates. To calculate her RAIA dose, she would first determine the supplemental dose needed to alleviate the pre-meal hyperglycemia. Since she is 100 mg/dL above her target, the correction would require 2 units of insulin (1 unit per 50 mg/dL). Additionally, to cover the glycemic excursion secondary to the meal, she would need 4 units of insulin, because each unit will cover 15 grams of carbohydrate intake. Therefore, a total dose of 6 units of RAIA would be appropriate to cover the pre-meal hyperglycemia and the glucose load imposed by the meal.
Other tools or methods may also be used to individualize or adjust insulin doses such as the 1500 rule and the 1800 rule.14 Further, other dose adjustment strategies may be implemented such as preemptive adjustments for the impact of exercise on glycemic levels.
Insulin stacking is a concept that should also be considered when dosing insulin. This occurs when a dose of insulin is injected in the presence of residual insulin from the previous dose.14 This is generally an issue with regular human insulin, but it can occur with any insulin in which residual stacking occurs with a resultant insulin concentration that causes hypoglycemia. Because of this stacking, RAIAs are usually administered at an interval of no less than 4 hours.14
SIDE EFFECTS OF INSULIN THERAPY
The principle side effects of insulin include weight gain, hypoglycemia, and lipohypertrophy.14 Lipohypertrophy, or the deposition of fat, is caused by the anabolic effect of insulin and may occur at injection sites. Lipohypertrophy may alter the absorption kinetics of insulin injected into affected areas and should be avoided. Lipohypertrophy may be circumvented by rotating injection sites. Weight gain is also associated with insulin therapy. This effect is variable and contingent on many factors. Hypoglycemia is a common side effect of insulin therapy and has been reviewed extensively.4 Generally, hypoglycemia is less common when a patient uses the correct dose of an insulin analog (instead of unmodified human insulin) with a PK profile that matches normal physiologic secretory patterns. The incidences of nocturnal hypoglycemia and significant hypoglycemia that requires the assistance of a third party have been reduced in clinical trials of RAIAs, most of which compared RAIAs to unmodified human insulin. The differences in hypoglycemia are probably due to a reduction in subcutaneous residence time associated with RAIAs.15
INSULIN AND TECHNOLOGIES IN DEVELOPMENT
The currently available RAIAs provide PK profiles that are superior to regular human insulin, but these formulations do not mirror normal physiologic insulin secretory patterns. Therefore, there is continued interest in developing ultra-rapid-acting insulin formulations and delivery platforms.
Inhaled insulin (Afrezza)
A recent innovation is a new form of inhaled insulin (Afrezza), which is currently on the market. Compared to injected insulin aspart in patients with type 1 diabetes, Afrezza resulted in inferior A1C control, but it reduced hypoglycemia across all A1C groups.11,22 Initially, Afrezza was co-marketed by MannKind and Sanofi, but it is now marketed solely by MannKind.
BioChaperone
Eli Lilly and Adocia have joined forces to develop an ultra-rapid-acting insulin analog.23 This product's enhanced absorption is based on BioChaperone technology, which utilizes a physical complex with insulin lispro that increases the absorption rate of the insulin while protecting the insulin from enzymatic degradation. A study in patients with type 1 diabetes demonstrated that the product had more rapid absorption than insulin lispro, a higher peak concentration, and more rapid elimination.23 Both U-100 and U-300 formulations using BioChaperone technology are being evaluated.
FIAsp
Another ultra-rapid-acting insulin formulation that is currently being investigated by Novo Nordisk is FIAsp.23 This formulation combines insulin aspart, nicotinomide, and arginine. The mechanism of enhancement of absorption of this formulation is thought to be due to increased blood flow in the subcutaneous injection depot. Phase 3 trials in both type 1 and type 2 patients have been conducted.
Hyaluronidase and insulin
Another approach to enhancing the absorption rate of insulin is through the use of human hyaluronidase combined with insulin lispro.23 Hyaluronidase causes a disruption of hyaluronic acid in subcutaneous adipose tissue. This, in turn, facilitates a more rapid distribution of the locally injected insulin at the injection site. One study of patients with type 2 diabetes demonstrated better control of glycemic excursions with the combination of hyaluronidase and insulin lispro than with lispro alone.23
VIAject
One approach to creating an ultra-rapid-acting insulin is to formulate regular human insulin in a manner that promotes the monomeric form. This approach has been utilized by Biodel: their formulation (VIAject) combines EDTA, which chelates zinc and destabilizes the hexameric form of insulin, and citric acid, which affects surface charges.24 The net result is an insulin formulation that facilitates rapid monomerization and results in ultra-rapid absorption from the subcutaneous depot. A study comparing this novel formulation to insulin lispro in patients with type 1 diabetes concluded that VIAject had faster onset and faster absorption than insulin lispro.24 Another study compared the vascular effects of VIAject with insulin lispro and regular human insulin in patients with type 2 diabetes.25 This study concluded that VIAject reduced oxidative stress and improved endothelial function compared to both insulin lispro and regular human insulin.
CONCLUSIONS
Diabetes is a common metabolic disorder and the incidence of the disease continues to rise. There are myriad pharmacologic approaches to the management of both type 1 and type 2 diabetes, but insulin therapy remains an effective treatment modality. The goal of insulin replacement or supplementation therapy is to provide glycemic control without causing significant side effects. Because of their PK profiles, this is best accomplished with insulin analogs. Particularly, RAIAs are used to mitigate postprandial glycemic excursions. It is important to consider and control these postprandial glycemic excursions because they contribute significantly to the overall glycemic burden and to the development of diabetes-related complications. While the 3 currently available RAIAs (insulin lispro, insulin glulisine, and insulin aspart) and a rapid-acting inhaled insulin represent improvements over regular human insulin, further work is being focused on the development of ultra-rapid-acting insulin analogs that could offer superior PK profiles.
REFERENCES
- White JR. A brief history of the development of diabetes medications. Diabetes Spectr. 2014;27(2):82-86.
- Centers for Disease Control and Prevention. Number (in millions) of civilian, non-institutionalized persons with diagnosed diabetes, United States 1980-2014. http://www.cdc.gov/diabetes/statistics/prev/national/figpersons.htm. Updated December 1, 2015. Accessed June 22, 2016.
- American Diabetes Association. Statistics about diabetes. http://www.diabetes.org/diabetes-basics/statistics/. Updated April 1, 2016. Accessed June 22, 2016.
- Cryer PE. Hypoglycemia in Diabetes. 3rd ed. Alexandria, VA: American Diabetes Association; 2016.
- Van Belle TL, Coppieters KT, von Herrath MG. Type 1 diabetes: etiology, immunology, and therapeutic strategies. Physiol Rev. 2011;91(1):79-118.
- American Diabetes Association. Classification and diagnosis of diabetes. Diabetes Care. 2016;39(Suppl 1):S13-22.
- Bailey TS, Grunberger G, Bode BW, et al. American Association of Clinical Endocrinologists and American College of Endocrinology 2016 outpatient glucose monitoring consensus statement. Endocr Pract. 2016;22(2):231-261.
- American Diabetes Association. Glycemic targets. Diabetes Care. 2016;39(Suppl 1):S39-46.
- Monnier L, Lapinski H, Colette C. Contributions of fasting and postprandial plasma glucose increments to the overall diurnal hyperglycemia of type 2 diabetic patients. Diabetes Care. 2003;26(3):881-885.
- Riddle M, Umpierrez G, DiGenio A, et al. Contributions of basal and postprandial hyperglycemia over a wide range of A1C levels before and after treatment intensification in type 2 diabetes. Diabetes Care. 2011;34(12):2508-2514.
- American Diabetes Association. Approaches to glycemic treatment. Diabetes Care. 2016;39(Suppl 1):S52-59.
- Neumiller J. Practical Insulin. 4th ed. Alexandria, VA: American Diabetes Association; 2015.
- Campbell RK, Neumiller JJ. Insulins. in White JR, Campbell RK, eds. ADA/PDR Medications for the Treatment of Diabetes. Alexandria, VA: American Diabetes Association; 2011:39-63.
- Meah F, Juneja R. Insulin tactics in type 2 diabetes. Med Clin North Am. 2015;99(1):157-186.
- Home PD. The pharmacokinetics and pharmacodynamics of rapid-acting insulin analogues and their clinic consequences. Diabetes Obes Metab. 2012;14(9):780-788.
- Heinemann L, Heise T, Wahl LC, et al. Prandial glycaemia after a carbohydrate-rich meal in type 1 diabetic patients using the rapid acting insulin analogue [Lys(B28), Pro(B29)] human insulin. Diabet Med. 1996;13(7):625-629.
- Lindholm A, McEwen J, Riis AP. Improved postprandial glycemic control with insulin aspart. A randomized double-blind cross-over trial in type 1 diabetes. Diabetes Care. 1999;22(5):801-805.
- Rave K, Klein O, Frick AD, Becker RH. Advantage of premeal-injected insulin glulisine compared with regular human insulin in subjects with type 1 diabetes. Diabetes Care. 2006;29(8):1812-1817.
- Novolog [package insert]. Plainsboro, NJ: Novo Nordisk Inc.; 2015.
- Apidra [package insert]. Bridgewater, NJ: sanofi-aventis U.S. LLC; 2015.
- Humalog [package insert]. Indianapolis, IN: Lilly USA, LLC; 2015.
- MannKind Corporation. Briefing Document: Endocrinologic and Metabolic Drug Advisory Committee. AFREZZA (insulin human [rDNA origin]) inhalation powder. An ultra-rapid acting insulin treatment to improve glycemic control in adult patients with diabetes mellitus. http://www.fda.gov/downloads/AdvisoryCommittees/CommitteesMeetingMaterials/Drugs/EndocrinologicandMetabolicDrugsAdvisoryCommittee/UCM390865.pdf. Updated April 1, 2014. Accessed June 8, 2016.
- Cahn A, Miccoli R, Dardano A, Del Prato S. New forms of insulin therapies for the treatment of type 2 diabetes. Lancet Diabetes Endocrinol. 2015;3(8):638-652.
- Heinemann l, Nosek L, Flacke F, et al. U-100, pH-neutral formulation of VIAject(®): faster onset of action than insulin lispro in patients with type 1 diabetes. Diabetes Obes Metab. 2012;14(3):222-227.
- Forst T, Pfutzner A, Flacke F, et al. Postprandial vascular effects of VIAject compared with insulin lispro and regular human insulin in patients with type 2 diabetes. Diabetes Care. 2010;33(1):116-120.
Back to Top